In Class:
Question to Ponder
A beam of blue light has a wavelegnth of 400 nm, while a beam of
infrared light has a wavelength of 2000nm. What can you say about the
speed of the light waves in the two beams?
- a) The speed of the blue light is five times greater than the
speed of the infrared light.
- b) The speed of the blue light is the same as the speed of the
infrared light.
- c) The speed of the blue light is five times less than the
speed of the infrared light.
- d) You can't say anything about the speeds of these light beams
without knowing the frequencies of the light waves.
Einstein Mucks Things Up
- Until Einstein comes along, everyone's happy with light as a wave
of electromagnetic energy.
- He worries about the Photo-electric Effect.
- Shine light onto a piece of metal.
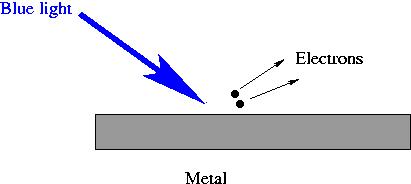
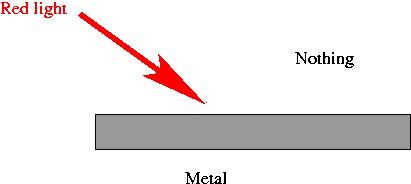
- When blue light is shined on the metal, electrons are knocked
off the metal.
- When red light is shined on the metal, nothing happens, even if
you use a really high intensity beam.
- It's the color (or more precisely the wavelength) of the light
that matters.
- Einstein concludes that light is composed of particles after all
(he calls them "photons").
- Blue light is able to knock the elecrons off the metal because
blue light particles have more energy per particle.
- Red light is made of photons of lower energy, each of which
doesn't have enough power to knock off and electron.
- A brighter red light just means more of those low energy photons,
but even in this case each individual photon doesn't have the energy
to knock an electron off.
- Einstein finds that the energy of a photon of light is related to
the wavelength as follows:
Energy of the photon = Eph = hc/lambda
where h is Planck's constant = 6.626 x 10-34 J s,
c is the speed of light = 3.00 x 108 m/s, and
lambda is the wavelength in units of m.
Wave-Particle Duality
- So in the end, we find that light seems to act like a wave
sometimes, and other times like a particle.
- In flight, light appears to behave very much like a wave. Young's
experiment on the diffraction and interference of light has been
repeated many times in many ways, and it's very clear that light does
things that plain old particles shouldn't do.
- But Einstein's experiment has also been repeated in a lot of
different ways, and it's also true that light interacts with matter in
a "quantized" way, delivering its energy in discrete packets whose
size depends on wavelength.
- The real problem here is with our insistence that light should
act like either a wave of a particle in the first place. Light just
acts like light. The fact that we don't have a good analogy for it
probably says more about the limitations of our imagination rather
than the quirky nature of light.
Making Light From Matter
- Light has to be generated somewhere, and most of the light we see
comes from matter.
- Light is nothing but a traveling packet of energy, so in order to
emit light, a material must give up some energy.
- Therefore, to be an emitter, a material must have "extra" energy
to give away, and a way to emit it as light.
- There are two general ways to do this (see below).
Thermal Emission: Making Light from Hot Stuff
- If you heat a dense material enough, it'll start to glow.
- It's not burning, but it is giving off light.
- The material is hotter than its surroundings, and it's cooling
off by shedding energy.
- Called "thermal," or "blackbody" emission, the light from a
heated object consists of a photons of a wide and continuous range of
wavelengths, e.g., its spectrum is a smooth, rainbow-like blur.
- The hotter the object gets, the more light is emitted.
- Also, the hotter the object gets, the more blue the emitted light
becomes; that is, the spectrum shifts to shorter wavelengths.
- This makes sense, since a hotter object will have more energy
available, and therefore can make more of the high energy (and short
wavelength) photons.
- Wien's Law describes how the peak of the spectrum (i.e., the
wavelength at which the emission is brightest) shifts with temperature
(see your book for a quantitative discussion).
- This is a remarkable result, because it allows us to determine the
temperature of an object simply by measuring the emission it produces.
Spectral Line Emission: Photons from Individual Atoms
- Thermal blackbody emission comes mainly from dense objects, where
the individual atoms are either well-linked to one another, or they're
so close together that they're always bumping into one another.
- In constrast, spectral line emission occurs when the density is
very low, and atoms are more-or-less free to roam about without
bumping into one another very often.
- In this case, emission occurs when an atom chages from a high
energy state to a low energy state.
- This can be visualized reasonably accurately with a simple atomic
model containing a small central nucleus containing neutrons and
protons surrounded by a cloud of orbiting electrons.
- Remarkably, the electrons can't fit into the atom is just any old
way; that is, there are only a few specific configurations that are
stable.
- You can think of the different configurations as electron orbits
that are closer to or farther from the nuclues (though this is a gross
simplification of real atomic structure).
- Each state of the atom has a certain specified energy, so when an
atom makes a change from one state to another lower energy state, it needs to
get rid of an amount of energy equal to the difference between the
energies of the two states.
- It gets rid of this "extra" energy by liberating a photon.
- Only photons whose energies are equal to the difference in energy between
two atomic states can be produced, so only a small set of specific
wavelength photons can be emitted by a particular atom.
- The result is a "line" spectrum consisting of emission only at a
few specific wavelengths, and no emission at other wavelengths.
- This means that the spectrum from each different kind of atom will
produce a different pattern of emission lines, and therefore produce
a kind of light "fingerprint" which is unique for each atomic
species.
- That means we can identify what elements are in stars or other
celestial objects just by looking at the pattern of emission in their spectra.
|